Guest blogger Richard I. Gregory, PhD, is a professor at Harvard Medical School and Principal Investigator in the Stem Cell Program at the Boston Children’s Hospital. His current research focuses on the epitranscriptome in cancer and his recent discoveries include the identification of RNA methyltransferases (MTases), METTL3 and METTL1, as new oncogenes in various cancer types.
—
Cellular RNAs are decorated with a diversity of chemical entities.1 The recent comprehensive mapping of sites of particular RNA chemical modifications has begun to define the epitranscriptome.2 Individual modifications occur on different subsets of coding and/or non-coding RNAs to influence their biogenesis, stability, localization, or function, and ultimately represent an important yet currently underappreciated aspect of post-transcriptional control of gene expression. Moreover, genetic mutation, amplification, and/or dysregulated expression of enzymes responsible for certain RNA modifications is linked to childhood developmental disorders and numerous types of cancer.
Transcriptome-Wide Approaches to Define the Global m7G RNA Methylome
N7-methylguanosine (m7G) is a prevalent tRNA modification in prokaryotes, eukaryotes, and some archaea that occurs at nucleotide position G46 in the variable loop of certain tRNAs. The modification helps to stabilize the functional conformation of tRNAs and thereby prevent their degradation.3
In the Gregory Laboratory at Boston Children’s Hospital, our recent studies have used antibody- and chemical-based approaches combined with high-throughput complementary DNA (cDNA) sequencing to comprehensively map m7G throughout the transcriptome. We developed m7G methylated tRNA immunoprecipitation sequencing (m7G MeRIP-Seq) and tRNA reduction and cleavage sequencing (TRAC-Seq) to specifically reveal the m7G tRNA methylome at single nucleotide resolution in mouse embryonic stem cells (mESCs) and human cancer cell lines.4-6 These global surveys uncovered the subset of tRNAs that are modified at a RAGGU sequence motif within the tRNA variable loop region.
These results also indicate that m7G modification is primarily restricted to tRNAs, a conclusion supported by related methods.7 Other contemporaneous studies, however, reported the presence of internal m7G modification in certain messenger RNAs (mRNAs) and primary microRNA (pri-miRNA) transcripts, though these findings are contentious and require further investigation.8-10
The m7G Methyltransferase (MTase) Complex: METTL1/WDR4
m7G46 is catalyzed by methyltransferase 1 (METTL1) and its essential cofactor, WD Repeat-Containing Protein 4 (WDR4), in mammals, which are orthologs of the previously identified Trm8/Trm82 complex in yeast.11 Recent structural studies provide new insight into how the m7G MTase complex specifically engages and modifies its specific subset of tRNA substrates.12, 13 The yeast complex is required for growth under heat stress but is not required under optimal conditions.14 In contrast, however, METTL1 and WDR4 are required for normal cell cycle progression and differentiation of mESCs and their mutation or dysregulation is linked to human disease.4, 15, 16
METTL1/WDR4 MTase Complex Deficiency Causes Developmental Defects
Germline mutations in the WDR4 gene, located at human chromosome 21q22.3, cause a distinct form of microcephalic primordial dwarfism characterized by facial dysmorphism, brain malformation, and severe encephalopathy with seizures.15, 16 WDR4 is also a candidate gene for some of the Down syndrome phenotypes, including mental retardation caused by trisomy of this chromosomal region in human patients.17 Indeed, WDR4 is one of a handful of candidate genes whose overexpression in mice influences learning and memory in a model of Down syndrome.18 Monosomy of the same cluster of genes also influences cognition and hippocampal plasticity, supporting the thesis that the precise dosage of certain gene(s) within this chromosomal region is critical for normal brain function.19
Interestingly, deletion of either METTL1 or WDR4 in mESCs causes cell differentiation defects, in particular, impaired ectoderm differentiation during neural lineage development (Figure 1).4 Mechanistically, ribosome profiling (Ribo-Seq) identified decreased translation efficiency of mRNAs related to the cell cycle and those controlling forebrain morphology in METTL1 knockout ESCs, and these mRNAs are enriched in codons that require m7G-modified tRNAs for translation.4
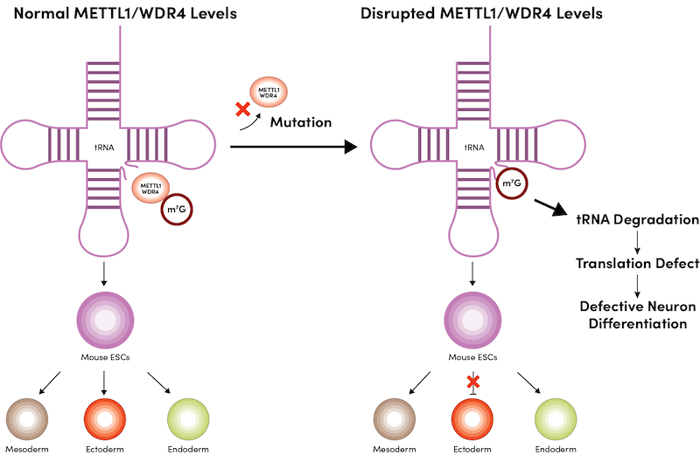
Figure 1: Deficiency of either METTL1 or WDR4 in mouse mESCs causes defective neuron differentiation.
Taken together, these findings on the molecular and cellular requirement for m7G tRNA modification in mESCs provide new insight into the etiology of these human developmental disorders.
METTL1/WDR4 MTase Complex Dysregulation Drives Cancer
We recently identified METTL1 as a potent new oncogene that is frequently amplified and/or overexpressed in many human cancer types.6 METTL1 deficiency strongly suppresses cancer cell proliferation, cell cycle progression, and tumor growth in several different cancer models.
Conversely, overexpression of the METTL1/WDR4 complex caused malignant transformation, including increased cell proliferation, accelerated cell cycle progression, enhanced colony formation, and in vivo tumor formation (Figure 2).
Figure 2: Elevated MTase complex expression leads to increased tRNA stability and abundance, causing increased translation of cell cycle regulator mRNAs, resulting in heightened cell growth and oncogenesis.
Mechanistically, elevated expression of the MTase complex led to the increased abundance of a subset of tRNAs that are m7G-modified and caused increased translation and protein expression of a subset of mRNAs, in particular, cell cycle regulators, which are enriched in codons that are decoded by m7G-modified tRNAs.6 The growing list of recent studies that highlight the importance of METTL1/WDR4 oncogenic activity in many different cancer types20-22 suggests that inhibition of this MTase complex could represent a promising new cancer therapeutic approach.
m7G tRNA Modification: Future Therapeutic Outlooks
As highlighted above, m7G modification occurs on a subset of tRNAs, stabilizes modified tRNAs, and is required for normal mRNA translation. Deficiency of the METTL1/WDR4 MTase complex leads to defective mouse stem cell self-renewal and neuronal differentiation, and is the genetic cause of certain human developmental disorders. Conversely, hyperactivation of the m7G MTase complex drives tumorigenesis. In both contexts, it is likely that changes in the functional tRNA pool and subsequent remodeling of the mRNA “translatome” is responsible for the observed phenotypes.
The overrepresentation of certain codons in distinct subsets of mRNAs and therefore the differential requirement for the corresponding sets of tRNAs—a phenomenon termed codon-biased translation, originally identified in stress response regulation in yeast—also implies that our current understanding of the genetic code is overly simplified. It suggests that certain tRNA modifications can have differential effects on mRNA translation and protein expression for certain subsets of genes.23
Developing a deeper understanding of the tRNA epitranscriptome and the role tRNA corruption plays in human disease could pave the way for novel therapeutic approaches targeting post-transcriptional gene regulation. For example, considering that METTL1 is oncogenic in many different types of cancer, and that genetic knockdown or knockout suppresses tumor growth in various mouse models, inhibiting this enzyme with small molecule drugs could represent a potential new cancer therapeutic strategy. The striking anti-cancer efficacy of a chemical inhibitor of the N6-methyladenosine (m6A) MTase, METTL3, in acute myelogenous leukemia (AML) mouse models further supports the promise of manipulating the epitranscriptome to treat cancer.24, 25 Moreover, in addition to its role in promoting cancer cell growth, METTL1 also influences the tumor microenvironment and therapeutic response to the immune checkpoint inhibitor (anti-PD-1 antibody) in a mouse intrahepatic cholangiocarcinoma (ICC) model.26 Identification of specific chemical inhibitors of METTL1 is now needed for the possible development of new and effective cancer treatments.
m7G is one of over 150 different known RNA modifications and there is rapidly growing interest in exploring the epitranscriptome more broadly. New methods and reagents that enable the precise mapping of individual modifications will be essential for the advancement of these research endeavors.
Additional Resources:
-
Learn more about Dr Gregory’s work on RNA modifications at Boston Children's Hospital.
-
Explore RNA regulatory mechanisms including RNA modifications.
-
Learn more about m6A, the most abundant mRNA modification in eukaryotes, in the guest blog post M6A: A Cell’s Hidden Signal of Gene Regulation by Gina Lee, PhD, Assistant Professor of Microbiology and Molecular Genetics at the University of California Irvine.
Select References:
-
Boccaletto P, Stefaniak F, Ray A, et al. MODOMICS: a database of RNA modification pathways. 2021 update. Nucleic Acids Res. 2022;50(D1):D231-D235. doi:10.1093/nar/gkab1083
-
Moshitch-Moshkovitz S, Dominissini D, Rechavi G. The epitranscriptome toolbox. Cell. 2022;185(5):764-776. doi:10.1016/j.cell.2022.02.007
-
Alexandrov A, Chernyakov I, Gu W, et al. Rapid tRNA decay can result from lack of nonessential modifications. Mol Cell. 2006;21(1):87-96. doi:10.1016/j.molcel.2005.10.036
-
Lin S, Liu Q, Lelyveld VS, Choe J, Szostak JW, Gregory RI. Mettl1/Wdr4-Mediated m7G tRNA Methylome Is Required for Normal mRNA Translation and Embryonic Stem Cell Self-Renewal and Differentiation. Mol Cell. 2018;71(2):244-255.e5. doi:10.1016/j.molcel.2018.06.001
-
Lin S, Liu Q, Jiang YZ, Gregory RI. Nucleotide resolution profiling of m7G tRNA modification by TRAC-Seq. Nat Protoc. 2019;14(11):3220-3242. doi:10.1038/s41596-019-0226-7
- Orellana EA, Liu Q, Yankova E, et al. METTL1-mediated m7G modification of Arg-TCT tRNA drives oncogenic transformation. Mol Cell. 2021;81(16):3323-3338.e14. doi:10.1016/j.molcel.2021.06.031
-
Marchand V, Ayadi L, Bourguignon-Igel V, Helm M, Motorin Y. AlkAniline-Seq: A Highly Sensitive and Specific Method for Simultaneous Mapping of 7-Methyl-guanosine (m7G) and 3-Methyl-cytosine (m3C) in RNAs by High-Throughput Sequencing. Methods Mol Biol. 2021;2298:77-95. doi:10.1007/978-1-0716-1374-0_5
-
Zhang LS, Liu C, Ma H, et al. Transcriptome-wide Mapping of Internal N7-Methylguanosine Methylome in Mammalian mRNA. Mol Cell. 2019;74(6):1304-1316.e8. doi:10.1016/j.molcel.2019.03.036
-
Pandolfini L, Barbieri I, Bannister AJ, et al. METTL1 Promotes let-7 MicroRNA Processing via m7G Methylation. Mol Cell. 2019;74(6):1278-1290.e9. doi:10.1016/j.molcel.2019.03.040
- Vinther J. No Evidence for N7-Methylation of Guanosine (m7G) in Human let-7e. Mol Cell. 2020;79(2):199-200. doi:10.1016/j.molcel.2020.05.022
- Alexandrov A, Martzen MR, Phizicky EM. Two proteins that form a complex are required for 7-methylguanosine modification of yeast tRNA. RNA. 2002;8(10):1253-1266. doi:10.1017/s1355838202024019
- Ruiz-Arroyo VM, Raj R, Babu K, Onolbaatar O, Roberts PH, Nam Y. Structures and mechanisms of tRNA methylation by METTL1-WDR4 [published online ahead of print, 2023 Jan 4]. Nature. 2023;10.1038/s41586-022-05565-5. doi:10.1038/s41586-022-05565-5
- Li J, Wang L, Hahn Q, et al. Structural basis of regulated m7G tRNA modification by METTL1-WDR4 [published online ahead of print, 2023 Jan 4]. Nature. 2023;10.1038/s41586-022-05566-4. doi:10.1038/s41586-022-05566-4
- Alexandrov A, Grayhack EJ, Phizicky EM. tRNA m7G methyltransferase Trm8p/Trm82p: evidence linking activity to a growth phenotype and implicating Trm82p in maintaining levels of active Trm8p. RNA. 2005;11(5):821-830. doi:10.1261/rna.2030705
- Filonava L, Torres AG, Ribas de Pouplana L. A novel cause for primordial dwarfism revealed: defective tRNA modification. Genome Biol. 2015;16:216. Published 2015 Oct 1. doi:10.1186/s13059-015-0786-y
- Shaheen R, Abdel-Salam GM, Guy MP, et al. Mutation in WDR4 impairs tRNA m(7)G46 methylation and causes a distinct form of microcephalic primordial dwarfism. Genome Biol. 2015;16:210. Published 2015 Sep 28. doi:10.1186/s13059-015-0779-x
- Michaud J, Kudoh J, Berry A, et al. Isolation and characterization of a human chromosome 21q22.3 gene (WDR4) and its mouse homologue that code for a WD-repeat protein. Genomics. 2000;68(1):71-79. doi:10.1006/geno.2000.6258
- Pereira PL, Magnol L, Sahún I, et al. A new mouse model for the trisomy of the Abcg1-U2af1 region reveals the complexity of the combinatorial genetic code of down syndrome. Hum Mol Genet. 2009;18(24):4756-4769. doi:10.1093/hmg/ddp438
- Sahún I, Marechal D, Pereira PL, et al. Cognition and hippocampal plasticity in the mouse is altered by monosomy of a genomic region implicated in Down syndrome. Genetics. 2014;197(3):899-912. doi:10.1534/genetics.114.165241
- Dai Z, Liu H, Liao J, et al. N7-Methylguanosine tRNA modification enhances oncogenic mRNA translation and promotes intrahepatic cholangiocarcinoma progression. Mol Cell. 2021;81(16):3339-3355.e8. doi:10.1016/j.molcel.2021.07.003
- Ma J, Han H, Huang Y, et al. METTL1/WDR4-mediated m7G tRNA modifications and m7G codon usage promote mRNA translation and lung cancer progression. Mol Ther. 2021;29(12):3422-3435. doi:10.1016/j.ymthe.2021.08.005
- Han H, Yang C, Ma J, et al. N7-methylguanosine tRNA modification promotes esophageal squamous cell carcinoma tumorigenesis via the RPTOR/ULK1/autophagy axis. Nat Commun. 2022;13(1):1478. Published 2022 Mar 18. doi:10.1038/s41467-022-29125-7
- Dedon PC, Begley TJ. Dysfunctional tRNA reprogramming and codon-biased translation in cancer. Trends Mol Med. 2022;28(11):964-978. doi:10.1016/j.molmed.2022.09.007
-
Yankova E, Blackaby W, Albertella M, et al. Small-molecule inhibition of METTL3 as a strategy against myeloid leukaemia. Nature. 2021;593(7860):597-601. doi:10.1038/s41586-021-03536-w
-
Li J, Gregory RI. Mining for METTL3 inhibitors to suppress cancer. Nat Struct Mol Biol. 2021;28(6):460-462. doi:10.1038/s41594-021-00606-5
-
Liu H, Zeng X, Ren X, et al. Targeting tumour-intrinsic N7-methylguanosine tRNA modification inhibits MDSC recruitment and improves anti-PD-1 efficacy [published online ahead of print, 2022 Oct 25]. Gut. 2022;gutjnl-2022-327230. doi:10.1136/gutjnl-2022-327230
Disclosures: Richard I. Gregory is a co-founder, scientific advisory board member, and equity holder of Redona Therapeutics, and receives compensation to participate in the Key Opinion Leader campaign from Cell Signaling Technology. The Gregory Lab receives or has received research funding from Sanofi, Astellas, and Ono.